A highly effective biological brake
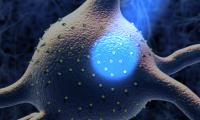
A team of scientists at the University of Copenhagen has turned movement ‘off‘ and ‘on’ in genetically modified laboratory mice by controlling specific brain cells. It is the first time this group of cells has been proven to have this function in mammals, a discovery that may have implications for understanding human brain disorders.
After more than five years of hard work in the lab, a team of researchers at the University of Copenhagen (UCPH) has identified a previously unknown function in the mammalian brain. Certain cells act as a ‘movement brake’, which turns the individual into a frozen statue in a split second.
The Lundbeck Foundation supported the project, and the discovery has just been announced in Nature Neuroscience, one of the most important brain research journals. Professor Ole Kiehn of the Department of Neuroscience at UCPH led the project. Two key colleagues were involved throughout the long process: Assistant Professor Roberto Leiras and Haizea Goni-Erro, PhD, the article’s first author.
The discovery was made with the help of optogenetics – the use of light to control brain-cell activity – in a particular area at the centre of the lab mice’s brains, called the pedunculopontine nucleus (PPN), which humans and other mammals also have. The mouse experiments focused on a small group of nerve cells in the PPN. What distinguishes these cells from others in this part of the brain is that they express the Chx10 gene.
The researchers genetically modified a line of lab mice, effectively building into their Chx10 cells an on/off function triggered by light (optogenetic activation). Small light sources – probes – were then inserted into the animals’ brains to flip this switch.
‘When the light shone directly on the Chx10 cells in the genetically modified mice, something very surprising happened,’ explains Professor Ole Kiehn, who also works at the Karolinska Institutet in Stockholm:
‘The mice stopped mid-movement. Their motor function just froze. The reaction occurred at lightning speed, within a fifth of a second. It shut down all motor activity and slowed their heart rate and breathing. A little later, when we turned off the light, and the nerve cells were no longer being artificially activated, the mice immediately resumed their physical activity.’
Remarkably, when they started moving again, they completed whatever movement had been interrupted when the scientists froze their motor function.
‘It was just like pausing a video while you make coffee and then pressing play when you’re back in the living room. The film just picks up where it left off. That’s why we call this phenomenon “pause-and-play”,’ Kiehn explains. The professor is an expert in the neuronal networks that control movement in mammals. In 2022, he was awarded the Lundbeck Foundation Brain Prize for his many years of research in this area.
Understanding illness
It is well known that in moments of abject fear, mammals, including humans, sometimes freeze and become rooted to the spot. The team stresses that this is not the same type of freezing described in the article in Nature Neuroscience:
‘Freezing induced by fear differs in several ways from the phenomenon we have studied,’ says Kiehn. ‘For example, when a mouse running across a field suddenly stiffens – because it senses a bird of prey hovering above – nerve cells from a completely different centre in the brain, the amygdala, are at play. If the mouse avoids being eaten, when it resumes motor activity, it doesn’t continue the same movement in which it was engaged before fear paralysed it. That isn’t a “pause-and-play” pattern – as we have seen in several fear-based animal experiments.’
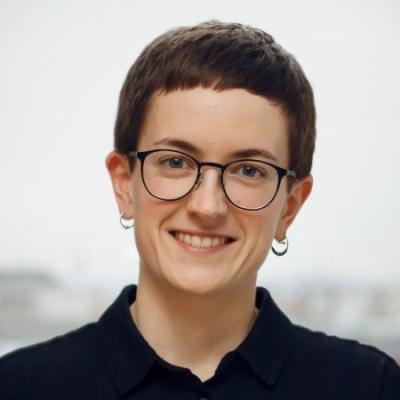
But if fear is not the catalyst, how do we explain the ‘pause-and-play’ phenomenon? And how might this discovery affect our understanding of illness and perhaps influence treatment?
According to the researchers involved, there is, as yet, no scientific explanation for the ‘pause-and-play’ effect.
‘But our hypothesis is that the pattern might be related to a form of hyper-sensitive focus on stimuli, such as sounds and smells in the outside world. In other words, these stimuli cause the small group of Chx10 nerve cells in the PNP to shut down motor activity completely – similar to the response we induced with light. We can’t explain why the motor activity resumes exactly where it left off. It may be related to information storage and processing in nerve-cell networks in the spinal cord, which control the musculoskeletal system in mammals. But that is something else we are still studying,’ says Kiehn.
As for the potential applications in terms of understanding and treating illness, Kiehn points – cautiously – to the neurodegenerative disorder Parkinson’s disease:
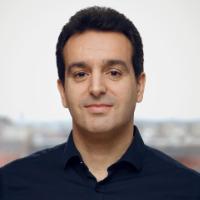
‘It’s not as if this discovery lends itself to any immediately tangible benefits in relation to Parkinson’s,’ he points out. ‘But it may be possible to extend our understanding of symptoms such as slowness and rigidity by taking a closer look at how the Chx10 nerve cells are activated in Parkinson’s patients. It may be the case that over-stimulation of the PPN nerve cells inhibits mobility.’
If this proves to be the case, the next logical step might be to develop a treatment that counteracts overactivity in Chx10 nerve cells in Parkinson’s patients. However, doing so with light via a probe operated into the patient’s brain raises all sorts of issues – including ethical ones.
‘It might be possible via some form of deep brain stimulation,’ Kiehn says.
Deep brain stimulation (DBS) is already used to treat Parkinson’s. It involves the insertion of an electrode into the brain, which delivers a weak current to selected brain regions. Among other things, the treatment aims to reduce slowness, rigidity and tremors in patients who do not respond well to existing medication.
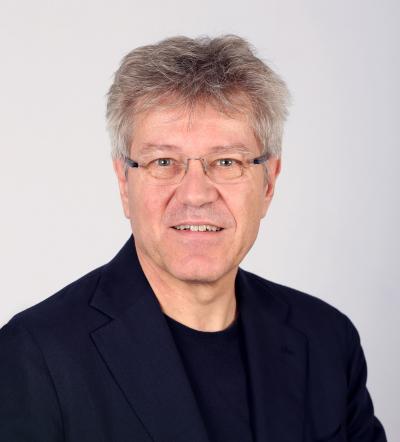
DBS experiments have shown that electrical stimulation can both trigger and inhibit cell activity. However, to influence the Chx10 nerve cells electrically, you must first figure out how to do so without affecting adjacent nerve cells. As Professor Kiehn explains, this presents a considerable challenge.
‘In our mouse experiments, we genetically modified the Chx10 nerve cells that we wanted to study so that they would react to the light, but the neighbouring cells would not. And you can’t do that in deep brain stimulation.’